Grade Level
All
minutes
15 min - 1 hr
subject
Physical Science
Activity Type:
data analysis, pressure experiments, pressure demonstrations, ocean exploration theme
Pressure is a Challenge to Deep Sea Explorers
Though oceans cover more than 70 percent of Earth’s surface, less than 5 percent of our oceans have been explored. Research and commercial vessels travel the surface of the ocean regularly, but the deepest parts of the ocean remain largely unseen by humans. The ocean floor in particular is home to unique marine organisms, as well as enormous geological features like volcanoes, canyons, and ridgelines. In order to take full advantage of these opportunities for discovery, scientists and explorers must first overcome one of the biggest challenges of deep ocean exploration: extreme pressure.
Pressure in the Deep
Everything in the deep ocean is under a great deal of pressure. At any depth in the ocean, the weight of the water above pushes on any object below it. With every foot an object descends into the ocean, more water is pushing down and against it, and more pressure is exerted upon that object. In fact, for every 10 meters traveled deeper into the ocean, there are an additional 6.47kg (14.27lbs) of pressure on each square inch of surface. In order to descend to greater ocean depths, scientists and explorers must use specially designed equipment like remotely operated vehicles (ROVs) and manned submersibles that can operate under extreme pressures. How extreme? An ROV diving to a depth of 2,000m experiences over 1,270kg (2,800lbs) of force exerted on each square inch of its surface!
Remembering Nereus, Explorer of Ocean Depths
Compressible Foam
A favorite pastime aboard exploration and research vessels like the exploration vessel (E/V) Nautilus is decorating foam cups to place atop ROVs during exploratory dives into the deep ocean (the authors of this educational resource traveled aboard the E/V Nautilus in 2015 as Science Communication Fellows). Aboard the E/V Nautilus, members of the Corps of Exploration stuff each foam cup with paper towels to help them keep their shape, then put the cups in a mesh bag tied to a milk crate attached to the ROV Argus that plunges into the ocean. After the exploratory dive is complete, the ROV is brought back up to the deck of the ship, and the cups are removed. Instead of returning to the surface the same size they went down, the cups are dramatically smaller, but weigh nearly the same amount. Why do the foam cups shrink?
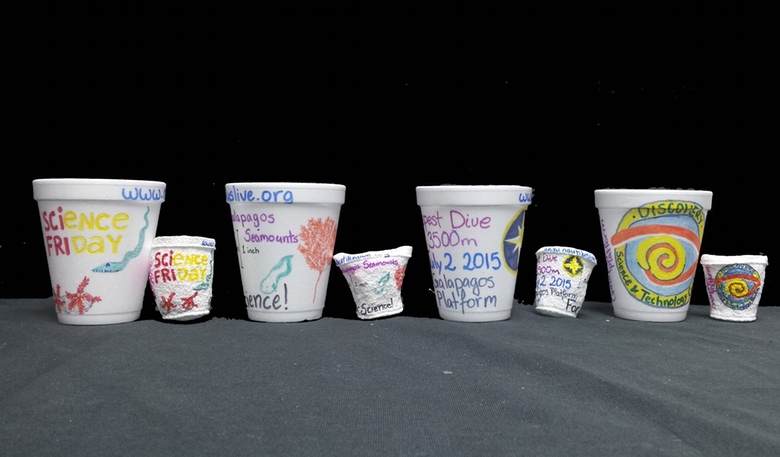
Foam cups are made from expanded polystyrene foam (EPF). Like most foam, EPF is made up of tiny polystyrene bubbles stuck together. When a foam cup descends into the ocean attached to an ROV, the air that fills its tiny polystyrene bubbles is compressed, and the polystyrene bubbles are crushed. At the bottom of the ocean, the pressure from the water is so great in all directions that the polystyrene beads are compressed uniformly, and the foam cups shrink to a fraction of their original size. At a depth of 2,000m, the pressure on a foam cup is equivalent to the weight of about 500 fully-grown giant Galápagos tortoises pressing down on it, so they stand no chance!
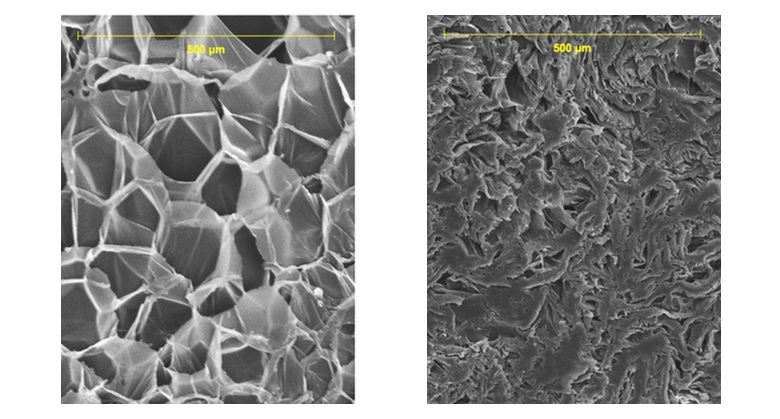
While compressing foam cups is fun for sea explorers, for fish and ROVs, compressibility is something to be avoided! The ROVs aboard the E/V Nautilus emerge from the depths the same size they went down because they are specially-designed using materials like aluminum, PVC, steel, and glass that are much, much less compressible than foam. Meanwhile, fish that travel to the deep ocean have specially-adapted bodies that are filled mostly with incompressible fluids instead of air, so that as they descend, their bodies retain their shape and size.
Simulate the Pressure Conditions of the Deep Ocean
Want to try your hand at pressure compaction at home? You can replicate the pressures of the deep ocean on EPF using a pump or syringe.
Safety note: Because of the high pressure generated in this activity, there is a risk that the bottle used may burst or detach from the pump. All observers should wear safety goggles and stay 4-6 feet from the demonstration. For added safety, have one person hold the bottle while a second uses the pump to add pressure.
Materials
2L soda bottle
2.5 cups polystyrene beads (or small marshmallows in a pinch)
Paper funnel
Bike pump or large plastic needleless syringe (>50 milliliter)
Duct tape or electrician’s tape
Permanent maker
Safety glasses
A helper
Water rocket pop-bottle connector, or other connector to form an airtight seal between the bottle and the pump. To download directions on how to make your own using a bike inner tube, bottle cap, and drill, click here: How to make a bike pump soda bottle adapter.
Simulate the Pressure Conditions of the Deep Ocean
Marshmallows, just like EPF cups, contain many small air bubbles, each with their own fixed amount of air pressure. In a low-pressure environment, the pressure inside the tiny air bubbles of a marshmallow (or foam object) is high enough to push outward against the ambient air, causing the bubbles to grow and the marshmallow to expand. But under high ambient pressure, the pressure of the air around the marshmallow is greater than the air trapped inside the marshmallow’s bubbles. As a result, the high-pressure ambient air pushes on the bubbles, compressing the air inside and causing them to shrink. Think of this pressure imbalance as a shoving contest between the air inside the foam and the air outside the foam: whichever has the most pressure will push more strongly and take up more space.
Extension: What happens when you shrink foam cups to different depths?
If pressure underwater increases with depth, then one might predict that if identical cups are sent down to deeper and deeper ocean depths, the cups that travel the deepest will shrink more than the cups that travel to shallower depths. In an original scientific experiment conducted aboard the E/V Nautilus, two members of the Nautilus Corps of Exploration decided to investigate that hypothesis. They created pairs of identical cups, and sent one from each pair to a different depth, leaving the second cup behind as an experimental control. To make it easier to quantitatively measure how much each cup shrank, a 5-centimeter scale was drawn on the side of each cup before it went down.
Each traveling cup descended aboard the ROV Argus to a unique depth—either 650m, 1180m, 1650m, 2200m, or 3300m. The final scale sizes of each cup are reported in the graph below:
Download data here: Raw Foam Cup Data
Use the data to answer the following questions:
- Is there a clear relationship between depth and how much each cup shrank?
- Take a look again at the SEM image of the shrunken foam at the top of this page. Does it provide any hints about the limits to which a foam cup can shrink?
- What do these data tell you about the depth at which the cup cannot shrink any more?
- Why might the amount of shrinkage differ if you were to measure different sides of the same cup? (Hint: Look at the pictures of the cups that were shrunken. How are they different?)
- Math challenge #1: What was the percentage decrease in cup size for each cup pair based on the scale measurements? What is the average percent decrease in the sizes of cups that traveled more than 600 m deep?
- Math challenge #2: Try to re-create this graph so that the x-axis is “pressure” rather than depth. Remember that for every 10 m deeper an ROV travels into the ocean, there is an additional 6.47kg (14.27lbs) of pressure on each square inch of surface.
About This Resource
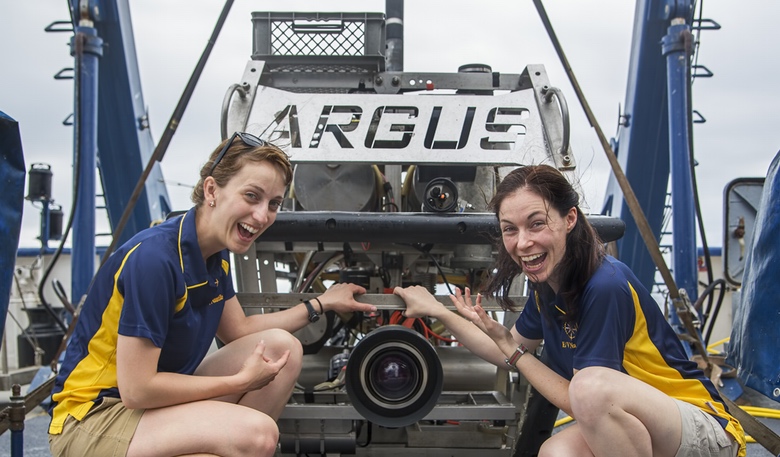
This resource was created by Rachel Rayner and Ariel Zych inspired by their experience as 2015 Science Communication Fellows aboard the Exploration Vessel (E/V) Nautilus during its July 2015 expedition to the Galápagos Islands. The Science Communication Fellowship Program, an initiative of the Ocean Exploration Trust, brings formal and informal educators together from around the world to support education aboard the E/V Nautilus as members of the Nautilus Corps of Exploration. Fellows are charged with the responsibility of engaging students and the public in the wonders of ocean exploration, sharing discoveries from missions as well as aspects of daily life aboard a working exploration vessel. To learn more about free opportunities for students and teachers to travel aboard the E/V Nautilus as members of the Corps of Exploration visit the Ocean Exploration Trust at www.oceanexplorationtrust.org.
About the Ocean Exploration Trust
The Ocean Exploration Trust was founded in 2008 by Dr. Robert Ballard to explore the ocean, seeking out new discoveries in the fields of geology, biology, maritime history, archaeology, physics, and chemistry while pushing the boundaries of STEM education and technological innovation. The Ocean Exploration Trust’s international program is launched from aboard the E/V Nautilus, offering live exploration to participants on shore and the public via live video, audio, and data feeds. Follow us online at www.nautiluslive.org, on Facebook and Instagram @NautilusLive, and on Twitter as @EVNautilus.
Educator's Toolbox
Meet the Writers
About Ariel Zych
@arieloquentAriel Zych is Science Friday’s director of audience. She is a former teacher and scientist who spends her free time making food, watching arthropods, and being outside.
About Rachel Rayner
Rachel Rayner is the Education Coordinator at the Discovery Science and Technology Centre, Bendigo, Victoria (Australia), where she designs educational resources, activities, workshops, shows and events for students and teachers.